PRACTICE SET THERMAL EXPANSION, CALORIMETRY & HEAT TRANSFER
2. PRACTICE SET-2
EXERCISE-2
THERMAL EXPANSION & CALORIMETRY
CALORIMETRY
Q.1 10 gm of ice at \(-20^{\circ} \mathrm{C}\) is added to \(10 \mathrm{gm}\) of water at \(50^{\circ} \mathrm{C}\). Specific heat of water \(=1 \mathrm{cal} / \mathrm{gm}-{ }^{\circ} \mathrm{C}\), specific heat of ice \(=0.5 \mathrm{cal} / \mathrm{gm}{ }^{\circ} \mathrm{C}\). Latent heat of ice \(=80 \mathrm{cal} / \mathrm{gm}\). Then resulting temperature is :-
(1) \(-20^{\circ} \mathrm{C}\)
(2) \(15^{\circ} \mathrm{C}\)
(3) \(0^{\circ} \mathrm{C}\)
(4) \(50^{\circ} \mathrm{C}\)
Q.2 Boiling water is changing into steam. Under this condition the specific heat of water is :-
(1) zero
(2) one
(3) infinite
(4) less than one
Q.3 Three liquids with masses \(\mathrm{m}_{1}, \mathrm{~m}_{2}, \mathrm{~m}_{3}\) are thoroughly mixed. If their specific heats are \(\mathrm{s}_{1}, \mathrm{~s}_{2}, \mathrm{~s}_{3}\) and their temperatures \(\theta_{1}, \theta_{2}, \theta_{3}\) respectively, then the temperature of the mixture is :-
(1) \(\frac{s_{1} \theta_{1}+s_{2} \theta_{2}+s_{3} \theta_{3}}{m_{1} s_{1}+m_{2} s_{2}+m_{3} s_{3}}\)
(2) \(\frac{m_{1} s_{1} \theta_{1}+m_{2} s_{2} \theta_{2}+m_{3} s_{3} \theta_{3}}{m_{1} s_{1}+m_{2} s_{2}+m_{3} s_{3}}\)
(3) \(\frac{m_{1} s_{1} \theta_{1}+m_{2} s_{2} \theta_{2}+m_{3} s_{3} \theta_{3}}{m_{1} \theta_{1}+m_{2} \theta_{2}+m_{3} \theta_{3}}\)
(4) \(\frac{m_{1} \theta_{1}+m_{2} \theta_{2}+m_{3} \theta_{3}}{s_{1} \theta_{1}+s_{2} \theta_{2}+s_{3} \theta_{3}}\)
Q.4 A stationary object at \(4^{\circ} \mathrm{C}\) and weighing \(3.5 \mathrm{~kg}\) falls from a height of 2000 metres on a snow mountain at \(0^{\circ} \mathrm{C}\). If the temperature of the object just before hitting the snow is \(0^{\circ} \mathrm{C}\) and the object comes to rest immediately (Take \(\mathrm{g}=10 \mathrm{~m} / \mathrm{s}^{2}\) ) and latent heat of ice is \(3.5 \times 10^{5} \mathrm{~J} / \mathrm{kg}\), then the object will melt :-
(1) 2 gm of ice
(3) 200 gm of ice
(2) 20 gm of ice
(4) \(2 \mathrm{~kg}\) of ice
Q.5 When vapour condenses into liquid :-
(1) it absorbs heat
(2) it liberates heat
(3) its temperature increases
(3) its temperature decreases
Q.6 A block of mass \(2.5 \mathrm{~kg}\) is heated to temperature of \(500^{\circ} \mathrm{C}\) and placed on a large ice block. What is the maximum amount of ice that can melt (approx.). Specific heat for the body \(=0.1 \mathrm{Cal} / \mathrm{gm}^{\circ} \mathrm{C}\).
(1) \(1 \mathrm{~kg}\)
(2) \(1.5 \mathrm{~kg}\)
(3) \(2 \mathrm{~kg}\)
(4) \(2.5 \mathrm{~kg}\)
Q.7 \(1 \mathrm{~kg}\) of ice at \(-10^{\circ} \mathrm{C}\) is mixed with \(4.4 \mathrm{~kg}\) of water at \(30^{\circ} \mathrm{C}\). The final temperature of mixture is : (specific heat of ice is \(2100 \mathrm{~J} / \mathrm{kg} / \mathrm{k}\) )
(1) \(2.3^{\circ} \mathrm{C}\)
(2) \(4.4^{\circ} \mathrm{C}\)
(3) \(5.3^{\circ} \mathrm{C}\)
(4) \(8.7^{\circ} \mathrm{C}\)
Q.8 Steam at \(100^{\circ} \mathrm{C}\) is added slowly to 1400 gm of water at \(16^{\circ} \mathrm{C}\) until the temperature of water is raised to \(80^{\circ} \mathrm{C}\). The mass of steam required to do this is \(\left(\mathrm{L}_{\mathrm{V}}=540 \mathrm{cal} / \mathrm{gm}\right)\) :
(1) \(160 \mathrm{gm}\)
(2) \(125 \mathrm{mg}\)
(3) \(250 \mathrm{gm}\)
(4) \(320 \mathrm{gm}\)
Q.9 A solid material is supplied with heat at a constant rate. The temperature of material is changing with heat input as shown in the figure. What does slope DE represent.
(1) latent heat of liquid
(2) latent heat of vapour
(3) heat capacity of vapour
(4) inverse of heat capacity of vapour
THERMAL EXPANSION, CALORIMETRY \& HEAT TRANSFER
Q.10 Ice at \(0^{\circ} \mathrm{C}\) is added to \(200 \mathrm{~g}\) of water initially at \(70^{\circ} \mathrm{C}\) in a vacuum flask. When \(50 \mathrm{~g}\) of ice has been added and has all melted the temperature of the flask and contents is \(40^{\circ} \mathrm{C}\). When a further \(80 \mathrm{~g}\) of ice has been added and has all metled, the temperature of the whole is \(10^{\circ} \mathrm{C}\). Calculate the specific latent heat of fusion of ice.[Take \(\mathrm{S}_{\mathrm{w}}=1 \mathrm{cal} / \mathrm{gm}{ }^{\circ} \mathrm{C}\).]
(1) \(3.8 \times 10^{5} \mathrm{~J} / \mathrm{kg}\)
(2) \(1.2 \times 10^{5} \mathrm{~J} / \mathrm{kg}\)
(3) \(2.4 \times 10^{5} \mathrm{~J} / \mathrm{kg}\)
(4) \(3.0 \times 10^{5} \mathrm{~J} / \mathrm{kg}\)
Q.11 A continuous flow water heater (geyser) has an electrical power rating \(=2 \mathrm{~kW}\) and efficienty of conversion of electrical power into heat \(=80 \%\). If water is flowing through the device at the rate of \(100 \mathrm{cc} / \mathrm{sec}\), and the inlet temperature is \(10^{\circ} \mathrm{C}\), the outlet temperature will be
(1) \(12.2^{\circ} \mathrm{C}\)
(2) \(13.8^{\circ} \mathrm{C}\)
(3) \(20^{\circ} \mathrm{C}\)
(4) \(16.5^{\circ} \mathrm{C}\)
Q.12 The graph shown in the figure represent change in the temperature of 5 \(\mathrm{kg}\) of a substance as it abosrbs heat at a constant rate of \(42 \mathrm{~kJ} \mathrm{~min}^{-1}\). The latent heat of vapourazation of the substance is :
(1) \(630 \mathrm{~kJ} \mathrm{~kg}^{-1}\)
(2) \(126 \mathrm{~kJ} \mathrm{~kg}^{-1}\)
(3) \(84 \mathrm{~kJ} \mathrm{~kg}^{-1}\)
(4) \(12.6 \mathrm{~kJ} \mathrm{~kg}^{-1}\)

Q.13 Find the amount of heat supplied to decrease the volume of an ice water mixture by \(1 \mathrm{~cm}^{3}\) without any change in temperature. \(\left(\rho_{\text {ice }}=0.9 \rho_{\text {water }}, \mathrm{L}_{\text {ice }}=80 \mathrm{cal} / \mathrm{gm}\right)\).
(1) \(360 \mathrm{cal}\)
(2) \(500 \mathrm{cal}\)
(3) \(720 \mathrm{cal}\)
(4) none of these
Q.14 Some steam at \(100^{\circ} \mathrm{C}\) is passed into \(1.1 \mathrm{~kg}\) of water contained in a calorimeter of water equivalent \(0.02 \mathrm{~kg}\) at \(15^{\circ} \mathrm{C}\) so that the temperature of the calorimeter and its contents rises to \(80^{\circ} \mathrm{C}\). What is the mass of steam condensing. (in \(\mathrm{kg}\) )
(1) \(0.130\)
(2) \(0.065\)
(3) \(0.260\)
(4) \(0.135\)
Q.15 \(10 \mathrm{gm}\) of ice at \(0^{\circ} \mathrm{C}\) is kept in a calorimeter of water equivalent \(10 \mathrm{gm}\). How much heat should be supplied to the apparatus to evaporate the water thus formed? (Neglect loss of heat)
(1) \(6200 \mathrm{cal}\)
(2) \(7200 \mathrm{cal}\)
(3) \(13600 \mathrm{cal}\)
(4) \(8200 \mathrm{cal}\)
Q.16 A \(2100 \mathrm{~W}\) continuous flow geyser (instant geyser) has water inlet temperature \(=10^{\circ} \mathrm{C}\) while the water flows out at the rate of \(20 \mathrm{~g} / \mathrm{sec}\). The outlet temperature of water must be about
(1) \(20^{\circ} \mathrm{C}\)
(2) \(30^{\circ} \mathrm{C}\)
(3) \(35^{\circ} \mathrm{C}\)
(4) \(40^{\circ} \mathrm{C}\)
Q.17 A block of ice with mass \(m\) falls into a lake. After impact, a mass of ice \(m / 5\) melts. Both the block of ice and the lake have a temperature of \(0^{\circ} \mathrm{C}\). If \(\mathrm{L}\) represents the heat of fusion, the minimum distance the ice fell before striking the surface is
(1) \(\frac{\mathrm{L}}{5 \mathrm{~g}}\)
(2) \(\frac{5 \mathrm{~L}}{\mathrm{~g}}\)
(3) \(\frac{g L}{5 m}\)
(4) \(\frac{m L}{5 g}\)
Q.18 Heat is being supplied at a constant rate to a sphere of ice which is melting at the rate of \(0.1 \mathrm{gm} / \mathrm{sec}\). It melts completely in \(100 \mathrm{sec}\). The rate of rise of temperature thereafter will be (Assume no loss of heat.)
(1) \(0.8^{\circ} \mathrm{C} / \mathrm{sec}\)
(2) \(5.4^{\circ} \mathrm{C} / \mathrm{sec}\)
(3) \(3.6^{\circ} \mathrm{C} / \mathrm{sec}\)
(4) will change with time
THERMAL EXPANSION
THERMAL EXPANSION, CALORIMETRY \& HEAT TRANSFER
Q.19 A steel tape gives correct measurement at \(20^{\circ} \mathrm{C}\). A piece of wood is being measured with the steel tape at \(0^{\circ} \mathrm{C}\). The reading is \(25 \mathrm{~cm}\) on the tape, the real length of the given piece of wood must be:
(1) \(25 \mathrm{~cm}\)
\((2)<25 \mathrm{~cm}\)
(3) \(>25 \mathrm{~cm}\)
(4) can not say
Q.20 If two rods of length \(\mathrm{L}\) and \(2 \mathrm{~L}\) having coefficients of linear expansion \(\alpha\) and \(2 \alpha\) respectively are connected so that total length becomes \(3 \mathrm{~L}\), the average coefficient of linear expansion of the composition rod equals:
(1) \(\frac{3}{2} \alpha\)
(2) \(\frac{5}{2} \alpha\)
(3) \(\frac{5}{3} \alpha\)
(4) none of these
Q.21 The bulk modulus of copper is \(1.4 \times 10^{11} \mathrm{~Pa}\) and the coefficient of linear expansion is \(1.7 \times 10^{-5}\left(\mathrm{C}^{\circ}\right)^{-1}\). What hydrostatic pressure is necessary to prevent a copper block from expanding when its temperature is increased from \(20^{\circ} \mathrm{C}\) to \(30^{\circ} \mathrm{C}\) ?
(1) \(6.0 \times 10^{5} \mathrm{~Pa}\)
(2) \(7.1 \times 10^{7} \mathrm{~Pa}\)
(3) \(5.2 \times 10^{6} \mathrm{~Pa}\)
(4) \(40 \mathrm{~atm}\)
Q.22 A rod of length \(20 \mathrm{~cm}\) is made of metal A. It expands by \(0.075 \mathrm{~cm}\) when its temperature is raised from \(0^{\circ} \mathrm{C}\) to \(100^{\circ} \mathrm{C}\). Another rod of a different metal B having the same length expands by \(0.045 \mathrm{~cm}\) for the same change in temperature, a third rod of the same length is composed of two parts one of metal A and the other of metal B. Thus rod expand by \(0.06 \mathrm{~cm}\).for the same change in temperature. The portion made of metal A has the length :
(1) \(20 \mathrm{~cm}\)
(2) \(10 \mathrm{~cm}\)
(3) \(15 \mathrm{~cm}\)
(4) \(18 \mathrm{~cm}\)
Q.23 A glass flask contains some mercury at room temperature. It is found that at different temperatures the volume of air inside the flask remains the same. If the volume of mercury in the flask is \(300 \mathrm{~cm}^{3}\), then volume of the flask is (given that coefficient of volume expansion of mercury and coefficient of linear expansion of glass are \(1.8 \times 10^{-4}\left({ }^{\circ} \mathrm{C}\right)^{-1}\) and \(9 \times 10^{-6}\left({ }^{\circ} \mathrm{C}\right)^{-1}\) respectively)
(1) \(4500 \mathrm{~cm}^{3}\)
(2) \(450 \mathrm{~cm}^{3}\)
(3) \(2000 \mathrm{~cm}^{3}\)
(4) \(6000 \mathrm{~cm}^{3}\)
Q.24 A thin copper wire of length \(\mathrm{L}\) increase in length by \(1 \%\) when heated from temperature \(\mathrm{T}_{1}\) to \(\mathrm{T}_{2}\). What is the percentage change in area when a thin copper plate having dimensions \(2 \mathrm{~L} \times \mathrm{L}\) is heated from \(\mathrm{T}_{1}\) to \(\mathrm{T}_{2}\) ?
(1) \(1 \%\)
(2) \(2 \%\)
(3) \(3 \%\)
(4) \(4 \%\)
Q.25 The coefficients of thermal expansion of steel and a metal \(\mathrm{X}\) are respectively \(12 \times 10^{-6}\) and \(2 \times 10^{-6}\) per \(^{\circ} \mathrm{C}\). At \(40^{\circ} \mathrm{C}\), the side of a cube of metal \(\mathrm{X}\) was measured using a steel vernier callipers. The reading was \(100 \mathrm{~mm}\). Assuming that the calibration of the vernier was done at \(0^{\circ} \mathrm{C}\), then the actual length of the side of the cube at \(0^{\circ} \mathrm{C}\) will be
(1) \(>100 \mathrm{~mm}\)
\((2)<100 \mathrm{~mm}\)
\((3)=100 \mathrm{~mm}\)
(4) data insufficient to conclude
Q.26 A rod of length \(2 \mathrm{~m}\) at \(0^{\circ} \mathrm{C}\) and having expansion coefficient \(\alpha=(3 x+2) \times 10^{-6 \circ} \mathrm{C}^{-1}\) where \(x\) is the distance (in cm) from one end of rod. The length of rod at \(20^{\circ} \mathrm{C}\) is :
(1) \(2.124 \mathrm{~m}\)
(2) \(3.24 \mathrm{~m}\)
(3) \(2.0120 \mathrm{~m}\)
(4) \(3.124 \mathrm{~m}\)
Q.27 A cuboid ABCDEFGH is anisotropic with \(\alpha_{x}=1 \times 10^{-5} /{ }^{\circ} \mathrm{C}\), \(\alpha_{y}=2 \times 10^{-5} /{ }^{\circ} \mathrm{C}, \alpha_{\mathrm{z}}=3 \times 10^{-5} /{ }^{\circ} \mathrm{C}\). Coefficient of superficial expansion of faces can be
(1) \(\beta_{\mathrm{ABCD}}=5 \times 10^{-5} /{ }^{\circ} \mathrm{C}\)
(3) \(\beta_{\mathrm{CDEH}}=3 \times 10^{-5} /{ }^{\circ} \mathrm{C}\)
(2) \(\beta_{\mathrm{BCGH}}=4 \times 10^{-5} /{ }^{\circ} \mathrm{C}\)
(4) \(\beta_{\mathrm{EFGH}}=2 \times 10^{-5} /{ }^{\circ} \mathrm{C}\)
THERMAL EXPANSION, CALORIMETRY \& HEAT TRANSFER
Q.28 A thin walled cylindrical metal vessel of linear coefficient of expansion \(10^{-3}{ }^{\circ} \mathrm{C}^{-1}\) contains benzene of volume expansion coefficient \(10^{-3 \circ} \mathrm{C}^{-1}\). If the vessel and its contents are now heated by \(10^{\circ} \mathrm{C}\), the pressure due to the liquid at the bottom.
(1) increases by \(2 \%\)
(2) decreases by \(1 \%\)
(3) decreases by \(2 \%\)
(4) remains unchanged
Q.29 An open vessel is filled completely with oil which has same coefficient of volume expansion as that of the vessel. On heating both oil and vessel,
(1) the vessel can contain more volume and more mass of oil
(2) the vessel can contain same volume and same mass of oil
(3) the vessel can contain same volume but more mass of oil
(4) the vessel can contain more volume but same mass of oil
Q.30 A solid ball of metal has a spherical cavity inside it. If the ball is heated the volume of the cavity will :
(1) increase
(2) decrease
(3) remain unchanged
(4) Date insufficient
Q.31 The reading of air thermometer at \(0^{\circ} \mathrm{C}\) and \(100^{\circ} \mathrm{C}\) are \(50 \mathrm{~cm}\) and \(75 \mathrm{~cm}\) of mercury column respectively. The temperature at which its reading is \(80 \mathrm{~cm}\) of mercury column is :
(1) \(105^{\circ} \mathrm{C}\)
(2) \(110^{\circ} \mathrm{C}\)
(3) \(115^{\circ} \mathrm{C}\)
(4) \(120^{\circ} \mathrm{C}\)
Q.32 On ehich of the following scales of temperature, the temperature is never negative :
(1) Celsium
(2) Fahrenheit
(3) Reaumur
(4) Kelvin
HEATTRANSFER
CONDUCTION
Q.33 A wall has two layer A and B each made of different material, both the layers have the same thickness. The thermal conductivity of the material A is twice that of \(\mathrm{B}\). Under thermal equilibrium the temperature difference across the wall \(\mathrm{B}\) is \(36^{\circ} \mathrm{C}\). The temperature difference across the wall \(\mathrm{A}\) is
(1) \(6^{\circ} \mathrm{C}\)
(2) \(12^{\circ} \mathrm{C}\)
(3) \(18^{\circ} \mathrm{C}\)
(4) \(72^{\circ} \mathrm{C}\)
Q.34 In the steady state of temperature the flow of heat across the body depends :
(1) only upon its thermal conductivity
(2) upon its thermal capacity
(3) upon its thermal conductivity and upon its thermal capacity
(4) neither upon its thermal conductivity nor upon its thermal capacity
Q.35 The rate of flow of heat through a metal bar of area of corss-section \(1 \mathrm{~m}^{2}\) when temperature gradient is \(1^{\circ} \mathrm{C} / \mathrm{m}\) under steady state is called
(1) thermal resistance
(2) thermal conductivity
(3) diffusibility
(4) resistivity
Q.36 In which case does the thermal conductivity increase from left to right
(1) \(\mathrm{Al}, \mathrm{Cu}, \mathrm{Ag}\)
(2) \(\mathrm{Ag}, \mathrm{Cu}, \mathrm{Al}\)
(3) \(\mathrm{Cu}, \mathrm{Ag}, \mathrm{Al}\)
(4) \(\mathrm{Al}, \mathrm{Ag}, \mathrm{Cu}\)
Q.37 Four rods with different radii \(\mathrm{r}\) and length \(\ell\) are used to connect two reservoirs of heat at different temperatures. Which one will conduct most heat?
(1) \(\mathrm{r}=1 \mathrm{~cm}, \ell=1 \mathrm{~m}\)
(2) \(\mathrm{r}=2 \mathrm{~cm}, \ell=2 \mathrm{~m}\)
(3) \(\mathrm{r}=1 \mathrm{~cm}, \ell=1 / 2 \mathrm{~m}\)
(4) \(\mathrm{r}=2 \mathrm{~cm}, \ell=1 / 2 \mathrm{~m}\)
THERMAL EXPANSION, CALORIMETRY \& HEAT TRANSFER
Q.38 Heat is flowing through two cylindrical rods of same material. The diameters of the rods are in the ratio \(1: 2\) and their lengths are in the ratio \(2: 1\). If the temperature difference between their ends is same, then the ratio of amounts of heat conducted through them per unit time will be :
(1) \(1: 1\)
(2) \(2: 1\)
(3) \(1: 4\)
(4) \(1: 8\)
Q.39 A rod of \(1 \mathrm{~m}\) length and area of cross-section \(1 \mathrm{~cm}^{2}\) is connected across two heat reservoirs at temperatures \(100^{\circ} \mathrm{C}\) and \(0^{\circ} \mathrm{C}\) as shown. The heat flow per second through the rod in steady state will be [Thermal conductivity of material of \(\operatorname{rod}=0.09\) kilocal \(\left.\mathrm{m}^{-1} \mathrm{~s}^{-1}\left({ }^{\circ} \mathrm{C}\right)\right]\)
(1) \(9 \times 10^{-4} \mathrm{kilocal} / \mathrm{sec}\)
(2) \(9 \mathrm{kilocal} / \mathrm{sec}\)
(3) \(0.09 \mathrm{kilocal} / \mathrm{sec}\)
(4) \(9 \times 10^{-6} \mathrm{kilocal} / \mathrm{sec}\)
Q.40 When two ends of a rod wrapped with cotton are maintained at different temperatures and after some time every point of the rod attains a constant temperature, then -
(1) Conduction of heat at different points of the rod stops because the temperature is not increasing.
(2) Rod is bad conductor of heat
(3) Heat is being radiated from each point of the rod
(4) Each point of the rod is giving heat to its neighbor at the same rate at which it is receiving heat.
Q.41 If \(\ell\) is the length and \(\mathrm{A}\) is the area of cross-section of a rod and \(\mathrm{K}\) is themal conductivity of material, then the thermal resistance is given by
(1) \(\frac{\mathrm{K} \ell}{\mathrm{A}}\)
(2) \(\frac{\ell}{\mathrm{KA}}\)
(3) \(\frac{\ell \mathrm{K}}{\mathrm{A}}\)
(4) \(\frac{\mathrm{A}}{\mathrm{K} \ell}\)
Q.42 Two rods are connected as shown. The rods are of same length and same cross sectional area. In steady state, the temperature \((\theta)\) of the interface will be -
(1) \(60^{\circ} \mathrm{C}\)
(2) \(73.3^{\circ} \mathrm{C}\)
(3) \(46.7^{\circ} \mathrm{C}\)
(4) \(37.3^{\circ} \mathrm{C}\)
Q.43 A slab consists of two parallel layers of two different materials of same thickness and having thermal conductivities \(\mathrm{K}_{1}\) and \(\mathrm{K}_{2}\). The equivalent thermal conductivity \(\mathrm{K}\) of the slab is
(1) \(\mathrm{K}_{1}+\mathrm{K}_{2}\)
(2) \(\frac{2 \mathrm{~K}_{1} \mathrm{~K}_{2}}{\mathrm{~K}_{1}+\mathrm{K}_{2}}\)
(3) \(\frac{\mathrm{K}_{1}+\mathrm{K}_{2}}{\mathrm{~K}_{1} \mathrm{~K}_{2}}\)
(4) \(\mathrm{K}_{1} \mathrm{~K}_{2}\)
Q.44 Two rods of length \(\mathrm{d}_{1}\) and \(\mathrm{d}_{2}\) and coefficients of thermal conductivities \(\mathrm{K}_{1}\) and \(\mathrm{K}_{2}\) are kept touching each other. Both have the same area of cross-section. The equivalent thermal conductivity is:
(1) \(\mathrm{K}_{1}+\mathrm{K}_{2}\)
(2) \(\mathrm{K}_{1} \mathrm{~d}_{1}+\mathrm{K}_{2} \mathrm{~d}_{2}\)
(3) \(\frac{K_{2} d_{1}+K_{1} d_{2}}{d_{1}+d_{2}}\)
(4) \(\frac{\mathrm{d}_{1}+\mathrm{d}_{2}}{\frac{\mathrm{d}_{1}}{\mathrm{~K}_{1}}+\frac{\mathrm{d}_{2}}{\mathrm{~K}_{2}}}\)
THERMAL EXPANSION, CALORIMETRY \& HEAT TRANSFER
Q.45 A cylinder of radius \(\mathrm{R}\) made of a material of thermal conductivity \(k_{1}\) is surrounded by a cylindrical shell of inner radius \(\mathrm{R}\) and outer radius \(2 \mathrm{R}\) made of a material of thermal conductivity \(\mathrm{k}_{2}\). The two ends of the combined system are maintained at different temperatures. There is no loss of heat from the cylindrical surface and the system is in steady state. The effective thermal conductivity of the system is
\((1) \mathrm{k}_{1}+\mathrm{k}_{2}\)
(2) \(\frac{\mathrm{k}_{1} \mathrm{k}_{2}}{\mathrm{k}_{1}+\mathrm{k}_{2}}\)
(3) \(\frac{1}{4}\left(\mathrm{k}_{1}+3 \mathrm{k}_{2}\right)\)
(4) \(\frac{1}{4}\left(3 \mathrm{k}+\mathrm{k}_{2}\right)\)
Q.46 The wall with a cavity consists of two layers of brick separated by a layer of air. All three layers have the same thickness and the thermal conductivity of the brick is much greater than that of air. The left layer is at a higher temperature than the right layer and steady state condition exists. Which of the following graphs predicts correctly the variation of temperature T with distance d inside the cavity?
(1)
(2)
(3)
(4)
Q.47 Three identical rods \(\mathrm{AB}, \mathrm{CD}\) and PQ are joined as shown. P and \(\mathrm{Q}\) are mid points of \(\mathrm{AB}\) and \(\mathrm{CD}\) respectively. Ends \(\mathrm{A}, \mathrm{B}, \mathrm{C}\) and \(\mathrm{D}\) are maintained at \(0^{\circ} \mathrm{C}, 100^{\circ} \mathrm{C}, 30^{\circ} \mathrm{C}\) and \(60^{\circ} \mathrm{C}\) respectively. The direction of heat flow in PQ is
(1) from \(P\) to \(Q\)
(2) from \(Q\) to \(P\)
(3) heat does not flow in PQ
(4) data not sufficient
Q.48 A composite rod made of three rods of equal length and cross-section as shown in the fig. The thermal conductivities of the materials of the rods are \(\mathrm{K} / 2,5 \mathrm{~K}\) and \(\mathrm{K}\) respectively. The end \(\mathrm{A}\) and end \(\mathrm{B}\) are at constant temperatures. All heat entering the face A goes out of the end B there being no loss of heat from the sides of the bar. The effective thermal conductivity of the bar is
(1) \(15 \mathrm{~K} / 16\)
(2) \(6 \mathrm{~K} / 13\)
(3) \(5 \mathrm{~K} / 16\)
(4) \(2 \mathrm{~K} / 13\).
Q.49 Figure shows three different arrangements of materials 1, 2 and 3 to form a wall. Thermal conductivities are \(\mathrm{k}_{1}>\mathrm{k}_{2}>\mathrm{k}_{3}\). The left side of the wall is \(20^{\circ} \mathrm{C}\) higher than the right side. Temperature difference \(\Delta T\) across the material 1 has following relation in three cases :
(A)
(B)
(C)
(1) \(\Delta \mathrm{T}_{\mathrm{a}}>\Delta \mathrm{T}_{\mathrm{b}}>\Delta \mathrm{T}_{\mathrm{c}}\)
(3) \(\Delta \mathrm{T}_{\mathrm{a}}=\Delta \mathrm{T}_{\mathrm{b}}>\Delta \mathrm{T}_{\mathrm{c}}\)
(2) \(\Delta \mathrm{T}_{\mathrm{a}}=\Delta \mathrm{T}_{\mathrm{b}}=\Delta \mathrm{T}_{\mathrm{c}}\)
(4) \(\Delta \mathrm{T}_{\mathrm{a}}=\Delta \mathrm{T}_{\mathrm{b}}<\Delta \mathrm{T}_{\mathrm{c}}\)
Q.50 A \(2 \mathrm{~cm}\) thick slab of commercial thermocole, \(100 \mathrm{~cm}^{2}\) in cross-section and having thermal conductivity \(2 \times 10^{-4} \mathrm{cal} \mathrm{sec}^{-1} \mathrm{~cm}^{-1}\left(\mathrm{C}^{\circ}\right)^{-1}\) has insulating regions differing by \(100^{\circ} \mathrm{C}\). The quantity of heat flowing through it in a day will be \(-\)
(1) \(20.4 \mathrm{kcal}\)
(2) \(43.2 \mathrm{kcal}\)
(3) \(86.4 \mathrm{kcal}\)
(4) \(63.6 \mathrm{kcal}\)
THERMAL EXPANSION, CALORIMETRY \& HEAT TRANSFER
Q.51 The coefficient of thermal conductivity of copper is nine time that of steel. In the composite cylindrical bar shown in Fig. What will be the temp. at the junction of copper and steel?
(1) \(75^{\circ} \mathrm{C}\)
(2) \(67^{\circ} \mathrm{C}\)
(3) \(33^{\circ} \mathrm{C}\)
(4) \(25^{\circ} \mathrm{C}\)
Q.52 One end of a copper rod of length \(1.0 \mathrm{~m}\) and area of cross-sector \(10^{-3} \mathrm{~m}^{2}\) is immersed in boiling water and the other end in ice. If the coefficient of thermal conductivity of copper is \(92 \mathrm{cal} / \mathrm{ms}{ }^{\circ} \mathrm{C}\) and the latent heat of ice is \(8 \times 10^{4} \mathrm{cal} / \mathrm{kg}\), then the amount of ice which will melt in one minute is
(1) \(9.2 \times 10^{-3} \mathrm{~kg}\)
(2) \(8 \times 10^{-3} \mathrm{~kg}\)
(3) \(6.9 \times 10^{-3} \mathrm{~kg}\)
(4) \(5.4 \times 10^{-3} \mathrm{~kg}\)
Q.53 Two identical vessels, made of different materials having conductivities \(\mathrm{K}_{1}\) and \(\mathrm{K}_{2}\) are completely filled with ice at \(0^{\circ} \mathrm{C}\). Due to temperature of surroundings, the ice in the two vessels melts in 25 min and 20 min respectively. The ratio of \(\mathrm{K}_{1}\) and \(\mathrm{K}_{2}\) is
(1) \(5 / 4\)
(2) \(4 / 5\)
(3) \(16 / 25\)
(4) \(\frac{4 / 5}{\sqrt{(5 / 4)}}\)
Q.54 Two rods of length \(\ell\) and \(2 \ell\), thermal conductivities \(2 \mathrm{~K}\) and \(\mathrm{K}\) are connected end to end. If crosssectional areas of to rods are equal, then equivalent thermal conductivity of the system is
(1) \((5 / 6 /) \mathrm{K}\)
(2) \(1.5 \mathrm{~K}\)
(3) \(1.2 \mathrm{~K}\)
(4) \((8 / 9) \mathrm{K}\)
Q.55 Two rods of same length and material transfer given amount of heat in \(12 \mathrm{~s}\), when they are joined end to end. When they are joined length-wise then they will transfer same amount of heat in same conditions in
(1) \(1.5 \mathrm{~s}\)
(2) \(13 \mathrm{~s}\)
(3) \(24 \mathrm{~s}\)
(4) \(48 \mathrm{~s}\)
RADIATION
Q.56 A blackened steel plate is put in a dark room after being heated up to a high temperature. A white spot on the plate appears-
(1) brighter than the plate.
(2) as bright as the plate.
(3) dull as compared to the plate.
(4) appears to be yellow.
Q.57 The temperature of a black body becomes half of its original temperature, the amount of radiation emitted by the body will reduce to-
(1) \(1 / 16\)
(2) \(1 / 4\)
(3) \(1 / 2\)
(4) remains unchanged.
Q.58 At any temperature the radiation energy emitted by a cube of length \(\mathrm{L}\) is proportional to -
(1) \(\mathrm{L}\)
(2) \(\mathrm{L}^{2}\)
(3) \(\mathrm{L}^{3}\)
(4) None of the above
Q.59 Temperature of a piece of metal is increased from \(27^{\circ} \mathrm{C}\) to \(327^{\circ} \mathrm{C}\). The rate of emission of radiation by a metal will become -
(1) Double
(2) Four times
(3) Eight times
(4) Sixteen times
THERMAL EXPANSION, CALORIMETRY \& HEAT TRANSFER
Q.60 If the temperature of a black body is increased by \(50 \%\) then the amount of radiation emitted by it will-
(1) Increase by \(400 \%\)
(2) Decrease by \(400 \%\)
(3) Decrease by \(50 \%\)
(4) Increase by \(50 \%\)
Q.61 The effective area of a black body is \(0.1 \mathrm{~m}^{2}\) and its temperature is \(1000 \mathrm{~K}\). The amount of radiations emitted by it per min is -
(1) \(1.34 \mathrm{k}-\mathrm{cal}\)
(2) \(81 \mathrm{k}-\mathrm{cal}\)
(3) \(5.63 \mathrm{k}-\mathrm{cal}\)
(4) \(1.34 \mathrm{k}-\mathrm{J}\).
Q.62 A body at temperature \(\mathrm{T}(\mathrm{K})\) is kept in the surroundings at \(\mathrm{T}_{0}(\mathrm{~K})\). If \(\mathrm{T}>>\mathrm{T}_{0}\), then the rate of emission of heat by the body to the surroundings is proportional to-
(1) \(\left(\mathrm{T}-\mathrm{T}_{0}\right)^{4}\)
(2) \(\mathrm{T}^{4}-\mathrm{T}_{0}^{4}\)
(3) \(\left(\mathrm{T}-\mathrm{T}_{0}\right)^{1 / 4}\)
(4) \(\mathrm{T} / \mathrm{T}_{0}\)
Q.63 Radius of a sphere is \(\mathrm{R}\), density is \(\mathrm{d}\) and specific heat is \(\mathrm{s}\), It is heated and then allowed to cool. Its rate of decrease of temperature will be proportional to -
(1) \(\operatorname{Rds}\)
(2) \(1 / \mathrm{Rds}\)
(3) \(1 / \mathrm{R}^{2} \mathrm{ds}\)
(4) \(\mathrm{R}^{2} \mathrm{ds}\)
Q.64 If a body at \(27^{\circ} \mathrm{C}\) emits \(0.3\) watt of heat then at \(627^{\circ} \mathrm{C}\), it will emit heat equal to -
(1) \(24.3\) watt
(2) \(0.42\) watt
(3) \(2.42\) watt
(4) \(0.9\) watt
Q.65 A body takes 4 minutes to cool from \(100^{\circ} \mathrm{C}\) to \(70^{\circ} \mathrm{C}\). If the room temperature is \(15^{\circ} \mathrm{C}\) then how many minutes will it need to cool from \(70^{\circ} \mathrm{C}\) to \(40^{\circ} \mathrm{C}-\)
(1) 4
(2) 5
(3) 6
(4) 7
Q.66 A liquid takes 10 minutes to cool from \(80^{\circ} \mathrm{C}\) to \(50^{\circ} \mathrm{C}\). The temperature of the surroundings is \(20^{\circ} \mathrm{C}\). Assuming that the Newton's law of cooling is obeyed, the cooling constant will be -
(1) \(0.056 / \mathrm{mt}\)
(2) \(0.042 / \mathrm{mt}\)
(3) \(0.081 / \mathrm{mt}\)
(4) \(0.069 / \mathrm{mt}\)
Q.67 Radius of a calorimeter is \(\mathrm{r}\) and depth is \(\ell\). It is filled completely with water and then cooled from temperature \(\theta\) in the surroundings at a temperature \(\theta_{0}\).If the emissive power of the surface of calorimeter is 1 and that of open surface of water is \(0.5\), then the ratio of rates of heat radiated by the surface of calorimeter and open surface of water will be -
(1) \(\frac{\ell}{\mathrm{r}}\)
(2) \(1+\frac{\ell}{\mathrm{r}}\)
(3) \(1+\frac{2 \ell}{r}\)
(4) \(2\left(1+\frac{2 \ell}{r}\right)\)
Q.68 Temperatures of two hot bodies \(\mathrm{B}_{1}\) and \(\mathrm{B}_{2}\) are \(100^{\circ} \mathrm{C}\) and \(80^{\circ} \mathrm{C}\) respectively. The temperature of surrounding is \(40^{\circ} \mathrm{C}\). At \(\mathrm{t}=0\), the ratio of rates of cooling of the two bodies (liquid) \(\mathrm{R}_{1}: \mathrm{R}_{2}\) will be \(-\)
(1) \(3: 2\)
(2) \(5: 4\)
(3) \(2: 1\)
(4) \(4: 5\)
Q.69 Increasing the temperature of a black body -
(1) Frequency and wavelength both increase for maximum radiation.
(2) Frequency and wavelength both decrease for miximum radiation.
(3) Wavelength increases while frequency decreases for maximum radiation.
(4) Frequency increases while wavelength decreases for maximum radiation.
THERMAL EXPANSION, CALORIMETRY \& HEAT TRANSFER
Q.70 At \(700^{\circ} \mathrm{K}\) temperature, the wave length of maximum energy emitted by a body is \(4.08\) micron. If the temperature of body is increased to \(1400^{\circ} \mathrm{K}\), then the wavelength of maximum energy will be-
(1) \(10.2\) micron
(2) \(16.32\) micron
(3) \(8.16\) micron
(4) \(2.04\) micron
Q.71 Two stars A and B radiate maximum energy at wave lengths 4000 and 5000
respectivey. The ratio of their temperature will be -
(1) \(1: 2\)
(2) \(2: 1\)
(3) \(4: 5\)
(4) \(5: 4\)
Q.72 Wein's displacement law is shown by the following relation \(\lambda_{\mathrm{m}} \mathrm{T}=\mathrm{b}\) then the curve drawn between \(\log \mathrm{T}\) and \(\log \lambda_{\mathrm{m}}\) will be -
(3)
(4)
Q.73 The spectral emissive power \(\mathrm{E}_{\lambda}\) for a body at temperature \(\mathrm{T}_{1}\) is plotted against the wavelength and area under the curve is found to be A. At a different temperature \(T_{2}\) the area is found to be \(9 \mathrm{~A}\). Then \(\lambda_{1} / \lambda_{2}=\)
(1) 3
(2) \(1 / 3\)
(3) \(1 / \sqrt{3}\)
(4) \(\sqrt{3}\)
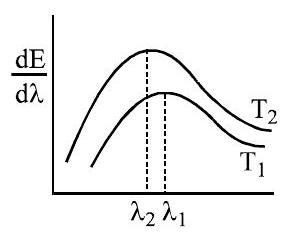
Q.74 A metal sphere cools from \(62^{\circ} \mathrm{C}\) to \(50^{\circ} \mathrm{C}\) in 10 minutes and then cools upto \(42^{\circ} \mathrm{C}\) in next 10 minutes. If the temperature of surrounding is \(26^{\circ} \mathrm{C}\), then its temperature after next 10 minutes will be-
(1) \(30^{\circ} \mathrm{C}\)
(2) \(34^{\circ} \mathrm{C}\)
(3) \(36.67^{\circ} \mathrm{C}\)
(4) \(38.52^{\circ} \mathrm{C}\)
Q.75 A black metal container is heated by radiation emitted by a small sphere at temperature \(\mathrm{T}\) and placed at a distance \(\mathrm{r}\). It is found that the power received by metal container is \(\mathrm{P}\). If the temperature and the distance are reduced to half their initial values, then the power received by metal container will be -
(1) \(\frac{P}{16}\)
(2) \(\frac{P}{4}\)
(3) \(\frac{P}{2}\)
(4) \(\mathrm{P}\)
Q.76 The intensity of heat radiation by a point source measured by a thermopile placed at a distance \(\mathrm{d}\) is I, If the distance of thermopile is doubled then the intensity of radiation will be -
(1) I
(2) \(2 \mathrm{I}\)
(3) \(\mathrm{I} / 4\)
(4) \(\mathrm{I} / 2\)
Q.77 If the experiment of Newton's law of cooling, the graph drawn between logarithm of temperature difference with surrounding the time is obtained as -
(1) A straight line passing through origin of positive gradient.
(2) A straight line of positive gradient, not passing through origin.
(3) A straight line of negative gradient not passing through origin.
(4) None of the above.
THERMAL EXPANSION, CALORIMETRY \& HEAT TRANSFER
Q.78 If the rates of loss of energy by unit area of an iron ball are \(\mathrm{E}_{1}, \mathrm{E}_{2}\) and \(\mathrm{E}_{3}\) when it cools from \(75^{\circ} \mathrm{C}\) to \(70^{\circ} \mathrm{C}, 70^{\circ} \mathrm{C}\) to \(65^{\circ} \mathrm{C}\) and \(65^{\circ} \mathrm{C}\) to \(60^{\circ} \mathrm{C}\) respectively then \(-\)
(1) \(\mathrm{E}_{1}=\mathrm{E}_{2}=\mathrm{E}_{3}\)
(2) \(\mathrm{E}_{1}>\mathrm{E}_{2}>\mathrm{E}_{3}\)
(3) \(\mathrm{E}_{1}<\mathrm{E}_{2}<\mathrm{E}_{3}\)
(4) \(\mathrm{E}_{1}<\mathrm{E}_{3}<\mathrm{E}_{2}\)
Q.79 The rate of emission of radiation of a black body at \(273^{\circ} \mathrm{C}\) is \(\mathrm{E}\), then the rate of emission of radiation of this body at \(0^{\circ} \mathrm{C}\) will be
(1) \(\frac{E}{16}\)
(2) \(\frac{E}{4}\)
(3) \(\frac{E}{8}\)
(4) 0
Q.80 Star \(\mathrm{S}_{1}\) emits maximum radiation of wavelength \(420 \mathrm{~nm}\) and the star \(\mathrm{S}_{2}\) emits maximum radiation of wavelength \(560 \mathrm{~nm}\), what is the ratio of the temperature of \(\mathrm{S}_{1}\) and \(\mathrm{S}_{2}\) :
(1) \(4 / 3\)
(2) \((4 / 3)^{1 / 4}\)
(3) \(3 / 4\)
(4) \((3 / 4)^{1 / 2}\)
Q.81 A black body calorimeter filled with hot water cools from \(60^{\circ} \mathrm{C}\) to \(50^{\circ} \mathrm{C}\) in 4 min and \(40^{\circ} \mathrm{C}\) to \(30^{\circ} \mathrm{C}\) in 8 min. The approximate temperature of surrounding is :
(1) \(10^{\circ} \mathrm{C}\)
(2) \(15^{\circ} \mathrm{C}\)
(3) \(20^{\circ} \mathrm{C}\)
(4) \(25^{\circ} \mathrm{C}\)
Q.82 From Kirchoff's law the ratio of emissive power and absorption power of all bodies -
(1) Are different.
(2) Is equal to emissive power of black body at same temperature.
(3) Is equal to emissive power of white body.
(4) Is equal to emissive power of black body at any temperature.